Looking for something?
Ligand Conjugation – Expanding the Reach of Oligonucleotide Therapeutics
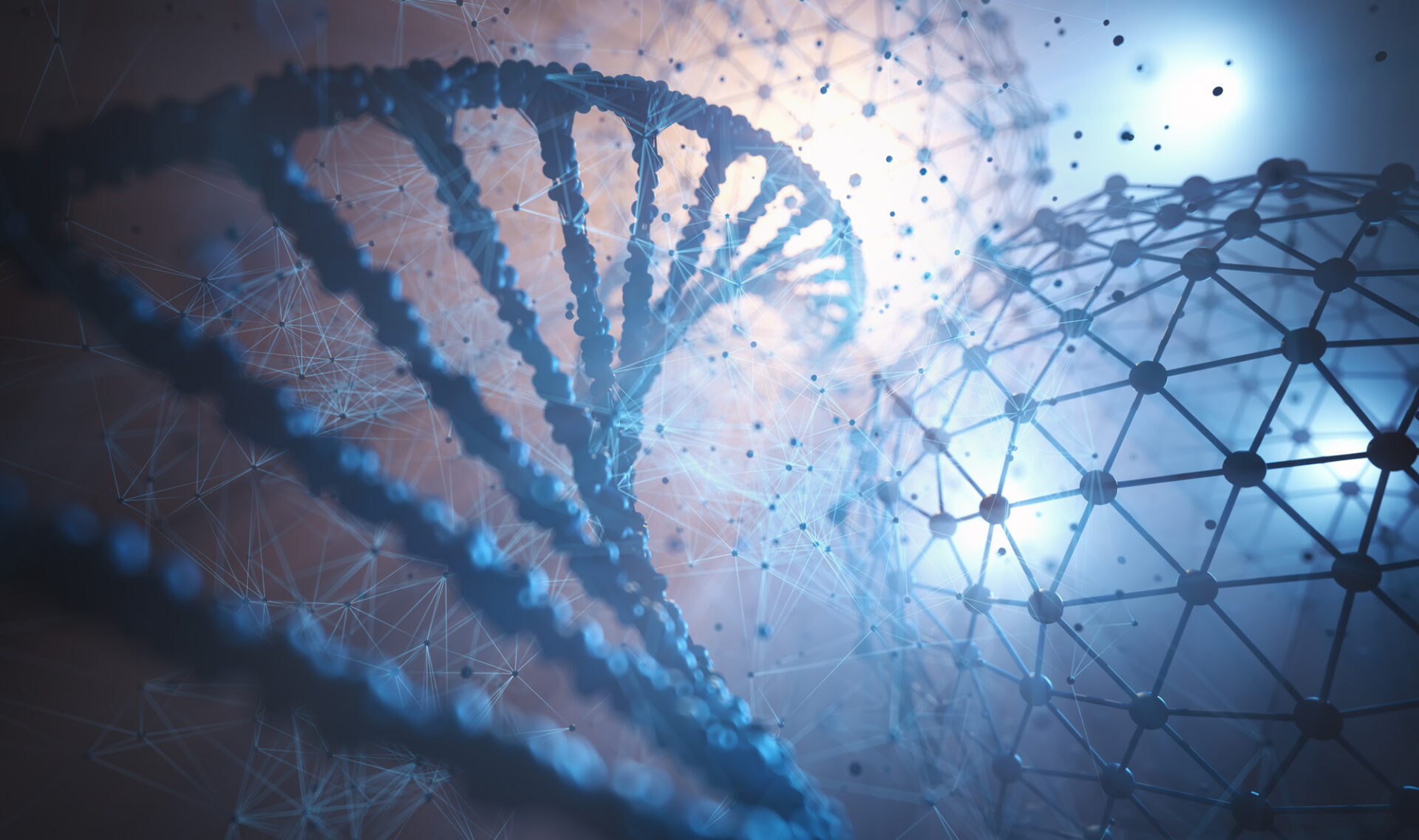
Oligonucleotide therapies possess enormous potential as healing, life-changing, and cancer-curing drugs. However, there are many barriers to utilizing oligonucleotides to treat disease. Discovering or creating effective delivery methods for each target cell or tissue type solves many of these problems and there are multiple solutions that have proven to work in some capacity. In a previous article, we delved into lipid-nanoparticles which have achieved tremendous success in delivering siRNAs, mRNA in COVID-19 vaccines, and a CRISPR treatment which is in clinical trials. Yet there is another delivery method that is just as promising and highly effective at delivering many types of oligonucleotides to various cell types – ligand conjugation.
Although there are many different ligands that could potentially be conjugated to oligonucleotides, ligand conjugation rests on an underlying principle. Each type of cell in the human body has specific molecules that can bind to the receptors on the cell. When these molecules (called ligands) are linked to an oligonucleotide, they facilitate delivery to cells that have the corresponding receptor. This allows for highly specific delivery to desired cell types, which minimizes drug exposure to other parts of the body and improves the safety of the drug. It also reduces the amount of drug required to treat disease. Many biomolecules have been investigated to this end.
One of the most well-known and successful conjugates is N-acetylgalactosamine (GalNAc), which binds to the asialoglycoprotein receptor (ASGPR) that is abundantly expressed in hepatocytes (the functional cells of the liver) (1, 2). GalNAc conjugation enables highly specific, efficient delivery of oligonucleotides to the liver. It also triggers potent and durable results (1, 3). Many companies have developed delivery platforms that utilize GalNAc conjugation.
Lipids, including cholesterol and fatty acids, may be an effective option to deliver oligonucleotides to the brain, liver, heart, kidneys, lungs, spleen, muscle, and adipose tissue. Cholesterol has been well studied (3) and one significant study in mice found that after a single intratumoral injection, cholesterol-conjugated, nuclease-resistant siRNAs achieved sustained and potent tumor-specific gene silencing (4). Docosahexaenoic acid may provide a solution to delivering siRNA to the brain (5) and conjugating docosanoic acid to siRNAs enabled efficient, sustainable, and non-toxic gene silencing in both skeletal and cardiac muscles after systemic injection (6). Palmitic acid conjugation improved the potency of ASOs in cardiac and skeletal muscle tissue (7).
Peptides can specifically interact with certain proteins on the cell surface (5) and may be useful in treating many diseases, including neuromuscular and neurodegenerative diseases (8, 9). Transferrin receptor 1-targeting peptides are being investigated to create low molecular weight ligands for muscle-specific targeted delivery of oligonucleotides. Folate is already used in delivery systems for drugs that fight cancer (10) because folate receptors are over-expressed in many tumors (5, 11). Transferrin is another ligand that could be used for the delivery of oligonucleotides to tumors, as well as the brain and endothelial cells (11).
Antibodies could be used whole or as fragments. They have high specificity and affinity for their targets (11). Conjugating siRNAs with anti-CD71 Fab’ fragment resulted in durable gene-silencing in the heart and skeletal muscle of mice for one month after intravenous administration. A myostatin-targeting siRNA conjugated with anti-CD71 Fab’ fragment was used to treat a mouse model of peripheral artery disease, which resulted in significant silencing of myostatin, leading to increased muscle mass and improved running performance (20). Not only are they useful as therapeutic agents, but also for imaging and detection (21). Aptamers, similarly to antibodies, have high specificity and affinity for their targets. However, they have low immunogenicity and are much smaller than antibodies (11). Conjugating siRNA to aptamers contributes to accumulation in certain cell types and some aptamers can penetrate the blood-brain barrier (5).
Other molecules have also been studied. Amisamide is a high-affinity ligand of sigma receptors that are over-expressed on many types of cancer. Anandamide is a ligand of the vanilloid and cannabinoid receptors that are expressed in certain B-cells and neuronal cells. Carbachol is structurally similar to acetylcholine and binds to muscarinic and nicotinic receptors which can be targeted to treat neuronal disorders and COPD. α-Tocopherol is a type of vitamin E, and it shows inherent antioxidant, anti-inflammatory, immune-stimulatory, anti-cancer, and nephroprotective properties (10). Many of these molecules displayed promising results but are still in early stages of discovery.
As you can see, each ligand has unique traits that cause it to be a valid option to investigate for delivering oligonucleotide to a specific cell type. Two excellent reviews cover the various ligands in great depth: Cellular Targeting of Oligonucleotides by Conjugation with Small Molecules and Current Development of siRNA Bioconjugates: From Research to the Clinic.
Although many groups have focused on conjugating a single type of molecule to an oligonucleotide, others have gone beyond this. One group recently experimented with designing a series of cholesterol-GalNAc dual conjugated ASOs and successfully reduced the renal distribution of ASOs while only slightly reducing gene-silencing activity which could reduce the nephrotoxic potential of ASOs (12).
Ligand conjugation has been engineered into delivery platforms with commercial applications and teams are racing to find effective ligand conjugations.
Alnylam Pharmaceuticals uses both lipid nanoparticles and conjugates to deliver siRNA. Alnylam designed a platform that conjugates three GalNAc molecules to one siRNA (trivalent GalNAc-conjugated siRNAs) to deliver siRNA to the liver and developed the enhanced stabilization chemistry (ESC)-GalNAc delivery platform which is still used today. Although other candidates were progressing and Patisiran reached commercial application, upon discovering that GalNAc-siRNA conjugate delivery platform resulted in far superior potency and safety, Alnylam stopped the other programs (2). Currently, Alnylam has three approved siRNA medicines, givosiran, lumasiran, and inclisiran (licensed to Novartis) which use this delivery platform, and many more in development to treat genetic, cardio-metabolic, and infectious diseases (2).
Alnylam and PeptiDream just announced an agreement to discover and develop peptide-siRNA conjugates to deliver siRNA to cell and tissue targets outside the liver. Alnylam will choose a set of receptors, then PeptiDream will use their peptide discovery platform to select, optimize, and synthesize peptides for each receptor. Alnylam will then create peptide-siRNA conjugates and perform studies to determine final peptide selection.
Arrowhead Pharmaceuticals developed a delivery platform called Dynamic PolyConjugates (DPCs) which employed GalNAc along with cholesterol modified siRNA (1, 2). Although candidates were in development with the DPC platform, when the company discovered a safer, more effective platform they had the courage to terminate studies that were in progress and moved to follow-on candidates that utilized the new platform, called Targeted RNAi Molecule (TRiM). TRiM can incorporate a variety of targeting ligands, including GalNAc and ligands of integrin αvβ3, αvβ5, and αvβ6 (2). With the ability to use ligands designed to transport siRNA to different types of cells, TRiM is being used to develop RNAi medicines that treat tumors and diseases in the lung, liver, and muscle, including hepatitis B, hypertriglyceridemia, and cystic fibrosis. The choice to switch to the new platform swiftly produced results and Arrowhead currently has multiple candidates in Phase 1 and 2 trials and partnerships with Janssen, Amgen, Takeda Pharmaceutical Company, and Horizon Therapeutics.
Dicerna Pharmaceuticals has also developed a GalNAc delivery platform for RNAi therapies. In contrast to Alnylam’s use of triantennary GalNAc conjugates, Dicerna uses four GalNAc molecules to create a tetra-antennary GalNAc conjugate (1, 2). This technology, the GalXC platform, has produced potent, durable effects. Dicerna is also developing technology to deliver siRNA beyond the liver. Since these technological advances retain key pharmacological features from GalXC, it is referred to ad GalXC-Plus, and may potentially be applied to skeletal muscle, central nervous system, and adipose tissue.
Silence Therapeutics is focused on targeting disease-associated genes in liver cells. In contrast to many other companies that are searching for methods to deliver oligonucleotides to tissues outside of the liver, Silence is committed to identifying new GalNAc mRNAi drugs to target many of the thousands of liver-expressed genes that have not yet been targeted. They have developed a GalNAc-siRNA platform called mRNAi GOLD. This platform is used in their medicine, SLN124, which is being developed to treat iron-loading anemia conditions, thalassemia, and myelodysplastic syndrome (MDS). Early results showed that SLN124 was well tolerated, improved red blood cell production, and reduced anemia. Another candidate, SLN360, was designed to silence the gene that makes lipoprotein(a), which is expected to lower the risk of heart attack, stroke, and heart disease.
Wave Life Sciences developed the stereopure chemistry platform which may allow for optimized pharmacological properties, including greater stability, enhanced activity and selectivity, and longer duration of effect, with broad applicability across modalities including antisense, exon-skipping, microRNA, RNAi, and RNA-guided gene editing (13). Currently focused on neurology, Wave Life Science has multiple candidates in the preclinical and clinical stages and collaborates with Takeda on four of them.
OliX Pharmaceuticals has developed asymmetric siRNA (asiRNA) to down-regulate expression of disease-causing genes. OliX partnered with AM Chemicals to combine AMC’s GalNAc-linker technology with OliX’s proprietary asymmetric siRNA (asiRNA) to develop therapies for liver diseases.
Regulus Therapeutics utilizes multiple strategies to deliver microRNA, and GalNAc conjugation is included among them. Regulus combines their proprietary linker conjugate with RNA technologies from Alnylam and Ionis (1). To treat disease, they develop mimics of microRNA as well as anti-miR, which inhibits microRNA. Regulus developed RG-101, a hepatocyte targeted GalNAc conjugated oligonucleotide that antagonizes miR-122 to treat Hepatitis C (14). RG-101 was recently investigated for use in combination with other drugs as a single-visit cure for chronic hepatitis C (15).
Geron has developed Imetelstat, an ASO conjugated to palmitic acid, targeting human telomerase to treat certain types of cancer. Geron is currently conducting two Phase 3 clinical trials of Imetelstat: IMerge, a Phase 2/3 trial in myelodysplastic syndromes (MDS), and IMpactMF, a Phase 3 trial in refractory myelofibrosis (MF).
Ionis Pharmaceuticals has developed three medicines that are commercially approved, with many more in clinical trials. Their newer platforms use ligand-conjugated antisense (LICA) technology to deliver ASOs to target cells. Following Alnylam’s lead that resulted in successful delivery of siRNA, Ionis conducted an in-depth investigation of triantennary GalNAc-conjugated ASOs. After determining the optimal structure, Ionis began adding THA-C6 triantennary GalNAc to ASO’s, which greatly increased their potency.
One example is AKCEA-TTR-LRX (ION-682884), a drug that shares the same nucleotide sequence as inotersen which has been approved for the treatment of adults with polyneuropathy of hereditary ATTR amyloidosis (hATTR). In contrast to inotersen, AKCEA-TTR-LRX is a ligand-conjugated antisense (LICA) drug that utilizes a triantennary N-acetylgalactosamine moiety (GalNAc3). This advanced design increases drug potency, which means that patients can be given lower, less frequent doses (16, 17). A Phase 3 clinical trial has begun to compare inotersen and AKCEA-TTR-LRX to determine the differences in patients’ neuropathy symptoms, quality of life, and TTR protein levels in the blood (18).
With multiple successes in delivering ASOs to the liver, Ionis has expanded their focus to identifying ligands that will enhance the delivery of ASOs to cells and tissues beyond the liver. So far, they have made significant progress in identifying ligands that enhance delivery to the pancreas, heart, and skeletal muscle.
In a collaboration between AstraZeneca and Ionis, it was determined that conjugating ASOs to a ligand of the glucagon-like peptide-1 receptor (GLP1R) productively delivers ASO cargo to pancreatic β-cells both in vitro and in vivo. The target genes in pancreatic islets were silenced without affecting target gene expression in the liver or other tissues (19).
Bicycle Therapeutics recently entered an agreement with Ionis that gives Ionis exclusive access to Bicycle’s proprietary bicyclic peptides (Bicycles) with high affinity to the transferrin receptor (TfR1) for the purpose of tissue-targeted delivery of oligonucleotide therapeutics. Bicycle does retain the rights to use TfR1 Bicycles for all non-oligonucleotide therapeutic purposes. The use of Bicycles expands the LICA platform, allowing muscle-specific targeted delivery with increased potency and more convenient administration, while reducing costs because manufacturing processes are less complex.
Suzhou Ribo Life Science Co. Ltd. (Ribo) is a leading siRNA drug development company in China that Ionis collaborated with to develop and commercialize RNA-targeted therapeutics in China. Ribo was granted a license for the right to commercialize Ionis Generation 2+ antisense drugs in metabolic disease, cancer, and possibly a third pre-specified Generation 2+ antisense drug. Ribo is also conducting a discovery program to identify drugs that utilize Ionis’ ssRNAi technology.
Ligand conjugation is proving to be a valuable approach to realizing the potential of oligonucleotides in a range of cell and tissue types throughout the body. Teams around the world are racing to engineer ligand conjugated oligonucleotide drugs with an ever-expanding range of targets to treat previously untreatable diseases and, although there has been significant progress in ligand conjugation technology, a lot of work still must be done to optimize new ligands and overcome potential safety concerns and potential adverse side effects. As countless hours are invested into this approach, we anticipate further extraordinary results that will leave a lasting impact.
References:
- Huang Y. (2017). Preclinical and Clinical Advances of GalNAc-Decorated Nucleic Acid Therapeutics. Molecular therapy. Nucleic acids, 6, 116–132. https://doi.org/10.1016/j.omtn.2016.12.003
- Hu, B., Zhong, L., Weng, Y., Peng, L., Huang, Y., Zhao, Y., & Liang, X. J. (2020). Therapeutic siRNA: state of the art. Signal transduction and targeted therapy, 5(1), 101. https://doi.org/10.1038/s41392-020-0207-x
- Osborn, M. F., & Khvorova, A. (2018). Improving siRNA Delivery In Vivo Through Lipid Conjugation. Nucleic acid therapeutics, 28(3), 128–136. https://doi.org/10.1089/nat.2018.0725
- Osborn, M. F., Coles, A. H., Golebiowski, D., Echeverria, D., Moazami, M. P., Watts, J. K., Sena-Esteves, M., & Khvorova, A. (2018). Efficient Gene Silencing in Brain Tumors with Hydrophobically Modified siRNAs. Molecular cancer therapeutics, 17(6), 1251–1258. https://doi.org/10.1158/1535-7163.MCT-17-1144
- Chernikov, I. V., Vlassov, V. V., & Chernolovskaya, E. L. (2019). Current Development of siRNA Bioconjugates: From Research to the Clinic. Frontiers in pharmacology, 10, 444. https://doi.org/10.3389/fphar.2019.00444
- Biscans, A., Caiazzi, J., McHugh, N., Hariharan, V., Muhuri, M., & Khvorova, A. (2021). Docosanoic acid conjugation to siRNA enables functional and safe delivery to skeletal and cardiac muscles. Molecular therapy: the journal of the American Society of Gene Therapy, 29(4), 1382–1394. https://doi.org/10.1016/j.ymthe.2020.12.023
- Prakash, T. P., Mullick, A. E., Lee, R. G., Yu, J., Yeh, S. T., Low, A., Chappell, A. E., Østergaard, M. E., Murray, S., Gaus, H. J., Swayze, E. E., & Seth, P. P. (2019). Fatty acid conjugation enhances potency of antisense oligonucleotides in muscle. Nucleic acids research, 47(12), 6029–6044. https://doi.org/10.1093/nar/gkz354
- Järver, P., Coursindel, T., Andaloussi, S. E., Godfrey, C., Wood, M. J., & Gait, M. J. (2012). Peptide-mediated Cell and In Vivo Delivery of Antisense Oligonucleotides and siRNA. Molecular therapy. Nucleic acids, 1(6), e27. https://doi.org/10.1038/mtna.2012.18
- Yin, H., Moulton, H. M., Seow, Y., Boyd, C., Boutilier, J., Iverson, P., & Wood, M. J. (2008). Cell-penetrating peptide-conjugated antisense oligonucleotides restore systemic muscle and cardiac dystrophin expression and function. Human molecular genetics, 17(24), 3909–3918. https://doi.org/10.1093/hmg/ddn293
- Hawner, M., & Ducho, C. (2020). Cellular Targeting of Oligonucleotides by Conjugation with Small Molecules. Molecules (Basel, Switzerland), 25(24), 5963. https://doi.org/10.3390/molecules25245963
- Yu, B., Zhao, X., Lee, L. J., & Lee, R. J. (2009). Targeted delivery systems for oligonucleotide therapeutics. The AAPS journal, 11(1), 195–203. https://doi.org/10.1208/s12248-009-9096-1
- Wada, F., Yamamoto, T., Ueda, T., Sawamura, M., Wada, S., Harada-Shiba, M., & Obika, S. (2018). Cholesterol-GalNAc Dual Conjugation Strategy for Reducing Renal Distribution of Antisense Oligonucleotides. Nucleic acid therapeutics, 28(1), 50–57. https://doi.org/10.1089/nat.2017.0698
- Apponi, L., Iwamoto, N., Frank-Kamenetsky, M., Yang, H., Braun, M., Shah, A., Butler, D., Lu, G., Vathipadiekal, V., Menon, S., Bowman, K., Pittet, L., Zhang, J., Zhong, Z., Vargeese, C., & Standley, S. (2018). Stereochemistry enhances pharmacological properties of APOC3 antisense oligonucleotides. Journal of Hepatology, 68.
- van der Ree, M. H. et al. Safety, tolerability, and antiviral effect of RG-101 in patients with chronic hepatitis C: a phase 1B, double-blind, randomised controlled trial. Lancet389, 709–717 (2017).
- Deng, Y., Campbell, F., Han, K., Theodore, D., Deeg, M., Huang, M., Hamatake, R., Lahiri, S., Chen, S., Horvath, G., Manolakopoulos, S., Dalekos, G. N., Papatheodoridis, G., Goulis, I., Banyai, T., Jilma, B., & Leivers, M. (2020). Randomized clinical trials towards a single-visit cure for chronic hepatitis C: Oral GSK2878175 and injectable RG-101 in chronic hepatitis C patients and long-acting injectable GSK2878175 in healthy participants. Journal of viral hepatitis, 27(7), 699–708. https://doi.org/10.1111/jvh.13282
- Crooke, S. T., Baker, B. F., Xia, S., Yu, R. Z., Viney, N. J., Wang, Y., Tsimikas, S., & Geary, R. S. (2019). Integrated Assessment of the Clinical Performance of GalNAc3-Conjugated 2′-O-Methoxyethyl Chimeric Antisense Oligonucleotides: I. Human Volunteer Experience. Nucleic acid therapeutics, 29(1), 16–32. https://doi.org/10.1089/nat.2018.0753
- Viney, N. J., Guo, S., Tai, L. J., Baker, B. F., Aghajan, M., Jung, S. W., Yu, R. Z., Booten, S., Murray, H., Machemer, T., Burel, S., Murray, S., Buchele, G., Tsimikas, S., Schneider, E., Geary, R. S., Benson, M. D., & Monia, B. P. (2021). Ligand conjugated antisense oligonucleotide for the treatment of transthyretin amyloidosis: preclinical and phase 1 data. ESC heart failure, 8(1), 652–661. https://doi.org/10.1002/ehf2.13154
- Coelho, T., Ando, Y., Benson, M. D., Berk, J. L., Waddington-Cruz, M., Dyck, P. J., Gillmore, J. D., Khella, S. L., Litchy, W. J., Obici, L., Monteiro, C., Tai, L. J., Viney, N. J., Buchele, G., Brambatti, M., Jung, S. W., St L O’Dea, L., Tsimikas, S., Schneider, E., Geary, R. S., … Gertz, M. (2021). Design and Rationale of the Global Phase 3 NEURO-TTRansform Study of Antisense Oligonucleotide AKCEA-TTR-LRx(ION-682884-CS3) in Hereditary Transthyretin-Mediated Amyloid Polyneuropathy. Neurology and therapy, 10(1), 375–389. https://doi.org/10.1007/s40120-021-00235-6
- Ämmälä, C., Drury, W. J., 3rd, Knerr, L., Ahlstedt, I., Stillemark-Billton, P., Wennberg-Huldt, C., Andersson, E. M., Valeur, E., Jansson-Löfmark, R., Janzén, D., Sundström, L., Meuller, J., Claesson, J., Andersson, P., Johansson, C., Lee, R. G., Prakash, T. P., Seth, P. P., Monia, B. P., & Andersson, S. (2018). Targeted delivery of antisense oligonucleotides to pancreatic β-cells. Science advances, 4(10), eaat3386. https://doi.org/10.1126/sciadv.aat3386
- Sugo T, Terada M, Oikawa T, Miyata K, Nishimura S, Kenjo E, Ogasawara-Shimizu M, Makita Y, Imaichi S, Murata S, Otake K, Kikuchi K, Teratani M, Masuda Y, Kamei T, Takagahara S, Ikeda S, Ohtaki T, Matsumoto H. Development of antibody-siRNA conjugate targeted to cardiac and skeletal muscles. J Control Release. 2016 Sep 10;237:1-13. doi: 10.1016/j.jconrel.2016.06.036. Epub 2016 Jun 29. PMID: 27369865.
- Dovgan I, Koniev O, Kolodych S, Wagner A. Antibody-Oligonucleotide Conjugates as Therapeutic, Imaging, and Detection Agents. Bioconjug Chem. 2019 Oct 16;30(10):2483-2501. doi: 10.1021/acs.bioconjchem.9b00306. Epub 2019 Jul 24. PMID: 31339691.