Looking for something?
Delivering Oligonucleotide Drugs to the Lungs in a World of Influenza, Coronaviruses, Asthma, and Chronic Lung Diseases
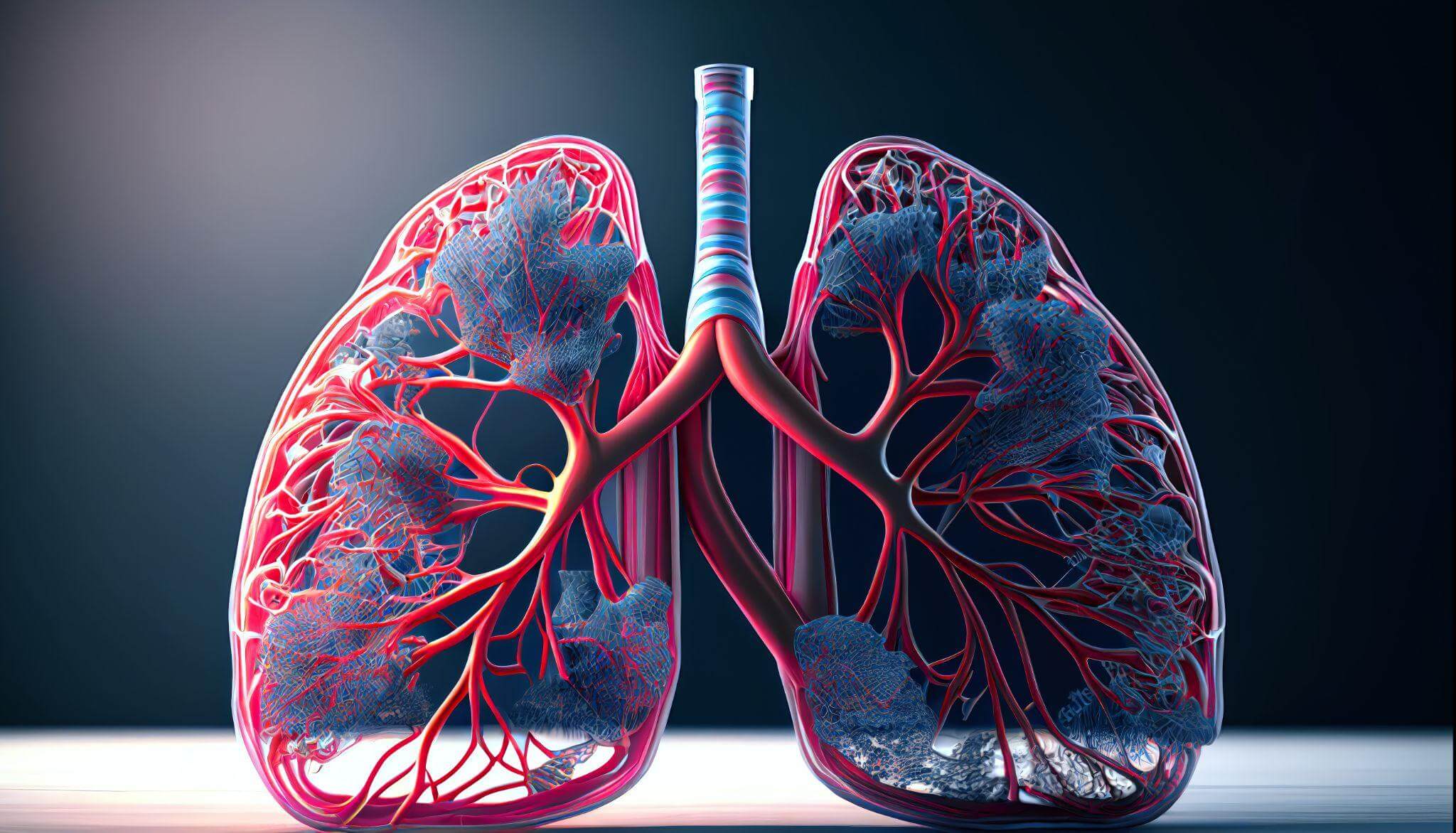
There are many different respiratory viruses, from influenza and respiratory syncytial virus (RSV) to coronaviruses, parainfluenza viruses, rhinoviruses, and adenoviruses. Some are highly pathogenic and can trigger respiratory failure and death. These viruses also facilitate secondary bacterial infections, often resulting in pneumonia, meningitis, and other acute inflammatory pathologies (1). These acute viral respiratory tract infections (AVRIs) are among the top five causes of death worldwide, with an average of 3.9 million lives lost each year, and the numbers soar higher when a virus such as H1N1 or SARS-CoV-2 causes a pandemic (1). Beyond AVRIs, other lung diseases such as cystic fibrosis, asthma, chronic obstructive lung disease (COPD), lung cancer, and even allergies pose a significant health burden and would benefit from a platform that provides non-invasive, localized delivery directly to the lungs.
Oligonucleotide therapeutics provide an excellent solution to meeting the unmet need for treatments for both respiratory viral infections and lung diseases because of their ability to target and interfere with the action of mRNA.
Yet, there are many barriers to lung delivery of oligonucleotide therapeutics. Despite the challenges, many teams are working on innovative solutions to safely deliver these drugs directly to the lungs. Recent reports indicate that a solution is imminent.
Is siRNA a Viable Option to Treat Lung Infections and Diseases?
Tissue distribution and stability are dependent upon the chemical architecture, conjugated ligand, and/or delivery vehicle, rather than the siRNA sequence (2). So, once a siRNA scaffold provides the desired pharmacokinetic properties for a specific tissue, the target sequence can be easily adapted (2). This makes siRNA an excellent strategy to inhibit viral RNA, and it is optimal since most respiratory viruses have an RNA genome, and synthetic siRNAs have been shown to effectively silence viral RNA (1). As viral infections depend upon the ability to replicate, if you can halt the replication process, you can treat the illness.
siRNA binds to mRNA and recruits endogenous cellular proteins to degrade the specific RNA target (2). As Jonathan K. Watts, PhD explained, “Using siRNA we can subvert the protein-production process by deleting specific disease-causing mRNA sequences before they are made into a protein, allowing us to treat the disease.”
Yet, systemically administered siRNAs face many challenges: they are degraded easily, cleared from the body quickly, and must cross cellular barriers and reach the intracellular site of action. In addition, they can produce unwanted side effects, including off-target activity and provoking an immune response (1). Although, it is interesting to note that it may be desirable to provoke an immune response when treating viral infections, and siRNA’s immunostimulatory activity can result in non-specific therapeutic effect (1).
Ideal siRNA delivery systems will condense siRNA into a stable particle, protect it from being degraded, improve cellular uptake, promote endosomal escape, and silence the target gene with high specificity while minimizing toxicity and off-target effects (3). Additionally, viruses mutate rather quickly, so it is vital to develop drugs that target viral genomic regions that are less likely to tolerate mutations (1).
Another interesting problem is that siRNA can accumulate in off-target tissues, but local delivery mitigates this problem. Many side effects can be minimized with a lower dose of siRNAs (1), and local delivery allows for lower doses. In the case of respiratory infections and lung diseases, pulmonary delivery would allow the optimal dose to be delivered directly to the target tissue, minimizing side effects and accumulation in off-target tissues.
Could Lung Delivery Be an Effective Solution?
Lungs are easily accessible for non-invasive, direct and localized administration of siRNA therapeutics (1). Pulmonary delivery allows the drug to reach all areas of the lung, as well as providing rapid absorption and onset of drug action and prolonged delivery periods (1, 4). Non-invasive lung delivery has the added benefit of allowing for self-medication (3).
Lung delivery allows for a smaller dose (1, 3) than is necessary with systemic administration, which has the dual benefit of reducing the risk of off-target activity and other unwanted side effects that are often associated with systemic injections (1).
However, the lungs are very sensitive to toxins and particles that can cause an immune response. Thus, the human body has many defenses to protect the lungs, including the intricate anatomy of the lungs, pulmonary surfactant, mucus and other host defenses that trap and remove particles from the airway, as well as macrophages that clear particles. These defenses create barriers to lung delivery (1, 3). Toxic effects are primarily related to inflammatory responses caused by particle-induced oxidative stress (3).
Many approaches have been tested to find an effective solution to overcoming these barriers. It has been determined that naked siRNA requires high doses that can cause toxicity, and viral vectors often cause severe immune responses (1). Chemical modifications and carrier molecules are potential solutions. Nanoparticles and lipid-based carriers have been explored as vehicles to deliver nucleic acid-based therapeutics to the lungs (3). As in subcutaneous injections with an LNP delivery system, nanoparticles encapsulate and protect the siRNA or mRNA from being degraded while facilitating cellular uptake. Once optimized for the target, nanoparticle delivery systems can be developed and brought to clinical trials with incredible speed (4).
Beyond finding efficient means of delivery, the route of administration must be considered when developing an oligonucleotide therapeutic. Pulmonary inhalation via nebulizers does require that the product be formulated specifically for this type of delivery. Particles that are too large can be deposited in the mouth and throat area, while particles that are too small will easily be exhaled or cleared quickly once in the body (1, 3).
Dry powder inhalation is another option for siRNA delivery, which may allow for extended shelf-life. For this to be successful, it must allow particles to be deposited near the target cells, and this can be achieved by optimizing the size, shape, and density of the particles (1).
Previous Work on Lung Delivery
In the early 2000s, many teams worked on antiviral siRNA therapeutics with a pulmonary delivery approach. Notably, Alnylam developed ALN-RSV01, which was a naked siRNA targeting RSV delivered via an intranasal spray. ALN-RSV01 was safe, well-tolerated, and 38% fewer people were infected. However, in the phase 2b study, it missed the primary endpoint and was abandoned (1).
Although this and other early studies were discontinued for various reasons, they laid the foundation for further avenues of study.
For example, in 2019, a group at Merck published a study demonstrating that chemically modified siRNA can mediate RNAi activity in multiple cells in the lung while avoiding immune system activation, but lung mRNA silencing is highly dependent on chemical modifications. They also demonstrated that intratracheally delivered siRNA resulted in target knockdown in lung cells. Further, the chemically modified siRNA did not induce inflammation or histological changes in the lung (5).
Another group of scientists at MIT published a study in 2019 showing a nanoparticle formulation that successfully delivered luciferase mRNA uniformly throughout the five lobes of mice lungs. Repeated dosing did not result in local or systemic toxicity (6).
Then, in 2020, the SARS-CoV-2 pandemic created an urgency for research and development of treatments for lung diseases.
Alnylam Pharmaceuticals (in partnership with Vir Biotechnology), Siranomics, and OliX Pharmaceuticals Inc. all began work to develop siRNA-based COVID-19 therapeutic or prophylactic candidates that would be formulated for intranasal administration or pulmonary inhalation (1).
Alnylam and Vir created a development candidate targeting the SARS-CoV-2 genome and planned to utilize Alnylam’s recent advances in lung delivery of siRNAs. Unfortunately, Alnylam and Vir’s candidate ALN-COV was discontinued due to “highly effective vaccines and alternative treatment options.”
Another recent study showed that distributing C16-siRNAs (conjugation of 2′-O-hexadecyl to siRNAs) to the lungs via intranasal administration to mouse lung tissue resulted in robust bronchiolar and alveolar uptake. A single 3 or 10 mg kg−1 dose administered intranasally provided greater reductions in Sod1 mRNA than a single 30 mg kg−1 dose given through an IV (7).
A New Platform Provides Broad Lung Distribution and Antiviral Activity
While many efforts have been abandoned, scientists at UMass Chan Medical School, have persisted and recently published a study demonstrating that chemically modified multimeric siRNA molecules were bioavailable in the lung after being intranasally or intratracheally administered and provided robust silencing in multiple lung cell types (2).
First, the team identified highly conserved SARS-CoV-2 regions for siRNA-based targeting, then designed and synthesized 108 fully chemically modified siRNAs and 53 LNA gapmer ASOs. Based on the results, they selected four siRNA lead compounds from different viral regions for more detailed characterization. They then evaluated the impact of siRNA multimerization and hydrophobic conjugation on lung delivery and distribution in order to identify the optimal scaffold for lung delivery. Mice were intratracheally dosed with the compounds, and lung distribution was evaluated 48 hours after administration (2).
The team discovered that “increasing the siRNA valency improved lung accumulation and overall distribution, while the increase in accumulation afforded by hydrophobic conjugation was less pronounced” (2). In order to test if the uptake was functional in the bulk lung, they targeted the housekeeping gene CD47 and found lung specific target silencing for all multivalent scaffolds one week after treatment (2).
At functional doses, there was no detectable toxicity, and the animals were healthy without observable adverse effects (2).
Local administration provided far higher absolute levels in the lung than systemic administration and was used as the route of administration for the subsequent experiments. Although robust silencing was achieved 2 weeks post administration, the team wanted to find a dosing regimen that provided higher silencing levels in a shorter time period (2).
As intratracheal administration is invasive and can produce significant inflammation at the injection site, they developed an intranasal administration model in which three doses of 10mg/kg divalent siRNA were administered three days apart, which provided robust levels of silencing of Cd47. This improvement allowed them to quickly test the efficacy of SAR-CoV-2 targeting siRNA in an infection model, reducing the time required for lead identification (2).
Two antiviral lead compounds were synthesized in a divalent scaffold, and mice were treated intranasally prior to SARS-CoV-2 infection. They found that “intranasal administration of divalent siRNA N_29293 demonstrated a profound impact on SARS-CoV-2 infection as evidenced by protection from weight loss, decreased viral plaques and viral mRNA abundance, and viral nucleocapsid detection by lung immunohistochemistry” (2). More uniform delivery was provided with multivalent architectures, as well as a 5- to 10-fold increase of accumulation in endothelial and alveolar cells. Robust silencing was observed in multiple lung cell types (2).
This is the first study to show that local administration of unformulated, fully chemically stabilized siRNA provides broad lung distribution and antiviral activity (2). It was mentioned that while intranasal and intratracheal administration was used in the rodent experiments, liquid nebulizers would need to be used to administer the oligonucleotides in larger animal models and clinical trials, to achieve uniform distribution beyond the upper respiratory tract (2). It was also mentioned that while the manuscript was being revised, the team also demonstrated that daily intranasal administration of LNA-modified ASOs potently suppresses SARS-CoV-2 replication in lungs of infected mice (2, 8).
To read the comprehensive description of the development and optimization of the siRNAs and LNA-ASOs, simply click on the following titles: Divalent siRNAs are bioavailable in the lung and efficiently block SARS-CoV-2 infection and An intranasal ASO therapeutic targeting SARS-CoV-2.
A New Class of Therapeutics
In light of the Covid-19 pandemic and many other respiratory viruses that have posed a major health concern over the past few decades, it has become increasingly apparent that we need therapeutics to treat acute viral respiratory tract infections. Oligonucleotide therapeutics are an optimal approach, as they can swiftly be adapted to target varying sequences.
As Vignesh N. Hariharan, PhD, co-author of the study and a postdoctoral associate in the lab of Anastasia Khvorova, PhD, said, “Achieving robust silencing at this level that is well tolerated hasn’t been achieved before. We’ve shown sufficient silencing to prove anti-viral efficacy can be done with siRNA and we think this architecture is the way forward to using RNA silencing therapeutically in the lungs. This would potentially open a new class of therapeutics for treating lung diseases.”
Even better, this approach could also be adapted to address other pulmonary diseases. That is precisely what the research team, which includes Drs. Anastasia Khvorova, Jonathan Watts, Vignesh Hariharan, and many other UMass Chan colleagues, is working to achieve, creating a platform that provides safe, efficient delivery to the lungs allowing specific disease-causing mRNA sequences to be deleted.
References:
- Mehta A, Michler T, Merkel OM. siRNA Therapeutics against Respiratory Viral Infections-What Have We Learned for Potential COVID-19 Therapies? Adv Healthc Mater. 2021 Apr;10(7):e2001650. doi: 10.1002/adhm.202001650. Epub 2021 Jan 27. PMID: 33506607; PMCID: PMC7995229.
- Hariharan VN, Shin M, Chang CW, O’Reilly D, Biscans A, Yamada K, Guo Z, Somasundaran M, Tang Q, Monopoli K, Krishnamurthy PM, Devi G, McHugh N, Cooper DA, Echeverria D, Cruz J, Chan IL, Liu P, Lim SY, McConnell J, Singh SP, Hildebrand S, Sousa J, Davis SM, Kennedy Z, Ferguson C, Godinho BMDC, Thillier Y, Caiazzi J, Ly S, Muhuri M, Kelly K, Humphries F, Cousineau A, Parsi KM, Li Q, Wang Y, Maehr R, Gao G, Korkin D, McDougall WM, Finberg RW, Fitzgerald KA, Wang JP, Watts JK, Khvorova A. Divalent siRNAs are bioavailable in the lung and efficiently block SARS-CoV-2 infection. Proc Natl Acad Sci U S A. 2023 Mar 14;120(11):e2219523120. doi: 10.1073/pnas.2219523120. Epub 2023 Mar 9. PMID: 36893269; PMCID: PMC10089225.
- Ding L, Tang S, Wyatt TA, Knoell DL, Oupický D. Pulmonary siRNA delivery for lung disease: Review of recent progress and challenges. J Control Release. 2021 Feb 10;330:977-991. doi: 10.1016/j.jconrel.2020.11.005. Epub 2020 Nov 9. PMID: 33181203.
- Shaffer C. Mist begins to clear for lung delivery of RNA. Nat Biotechnol. 2020 Oct;38(10):1110-1112. doi: 10.1038/s41587-020-0692-z. PMID: 33020636.
- Ng B, Cash-Mason T, Wang Y, Seitzer J, Burchard J, Brown D, Dudkin V, Davide J, Jadhav V, Sepp-Lorenzino L, Cejas PJ. Intratracheal Administration of siRNA Triggers mRNA Silencing in the Lung to Modulate T Cell Immune Response and Lung Inflammation. Mol Ther Nucleic Acids. 2019 Jun 7;16:194-205. doi: 10.1016/j.omtn.2019.02.013. Epub 2019 Feb 26. PMID: 30901578; PMCID: PMC6426712.
- Patel AK, Kaczmarek JC, Bose S, Kauffman KJ, Mir F, Heartlein MW, DeRosa F, Langer R, Anderson DG. Inhaled Nanoformulated mRNA Polyplexes for Protein Production in Lung Epithelium. Adv Mater. 2019 Feb;31(8):e1805116. doi: 10.1002/adma.201805116. Epub 2019 Jan 4. PMID: 30609147; PMCID: PMC7490222.
- Brown, K.M., Nair, J.K., Janas, M.M. et al. Expanding RNAi therapeutics to extrahepatic tissues with lipophilic conjugates. Nat Biotechnol (2022).
- Zhu C, Lee JY, Woo JZ, Xu L, Nguyenla X, Yamashiro LH, Ji F, Biering SB, Van Dis E, Gonzalez F, Fox D, Wehri E, Rustagi A, Pinsky BA, Schaletzky J, Blish CA, Chiu C, Harris E, Sadreyev RI, Stanley S, Kauppinen S, Rouskin S, Näär AM. An intranasal ASO therapeutic targeting SARS-CoV-2. Nat Commun. 2022 Aug 3;13(1):4503. doi: 10.1038/s41467-022-32216-0. PMID: 35922434; PMCID: PMC9349213.
“The views, opinions, findings, and conclusions or recommendations expressed in these articles and highlights are strictly those of the author(s) and do not necessarily reflect the views of the Oligonucleotide Therapeutics Society (OTS). OTS takes no responsibility for any errors or omissions in, or for the correctness of, the information contained in these articles. The content of these articles is for the sole purpose of being informative. The content is not and should not be used or relied upon as medical, legal, financial, or other advice. Nothing contained on OTS websites or published articles/highlights is intended by OTS or its employees, affiliates, or information providers to be instructional for medical diagnosis or treatment. It should not be used in place of a visit, call, consultation, or the advice of your physician or other qualified health care provider. Always seek the advice of your physician or qualified health care provider promptly if you have any healthcare-related questions. You should never disregard medical advice or delay in seeking it because of something you have read on OTS or an affiliated site.”