Looking for something?
The Astonishing History, Controversies, and Game-Changing Uses of CRISPR
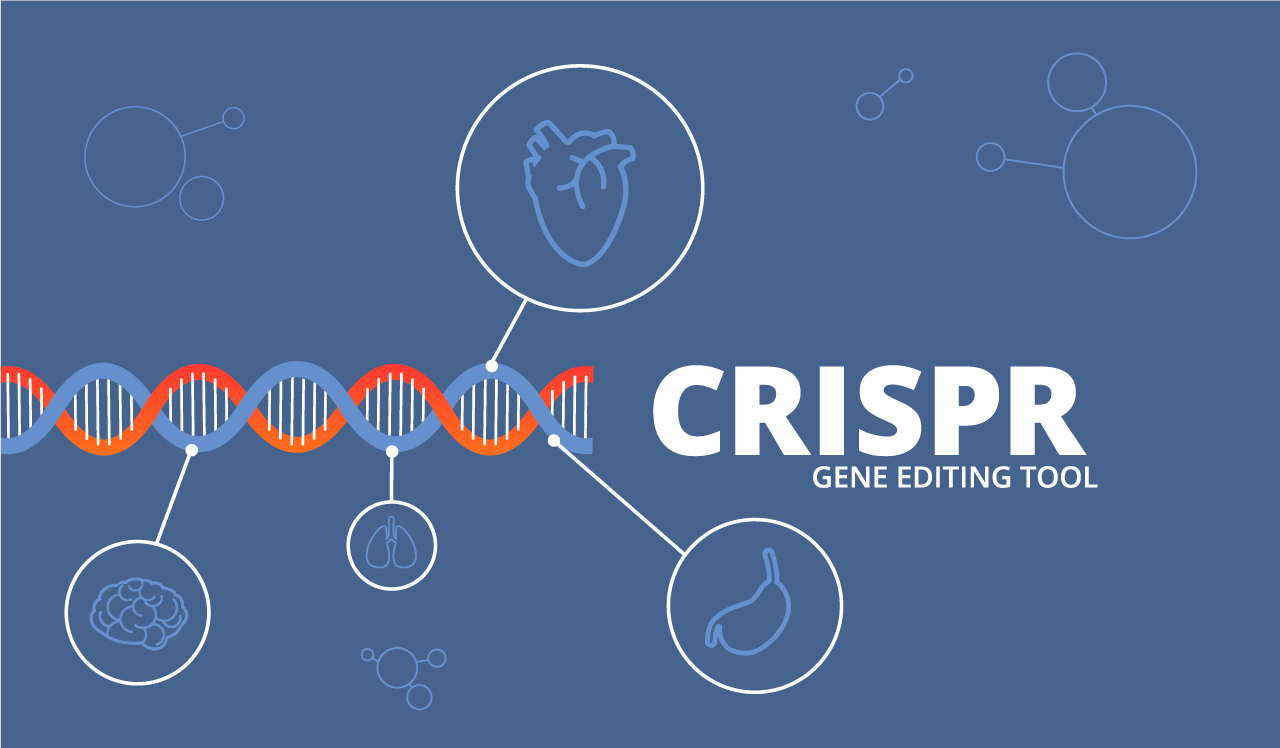
Many of us view CRISPR as an incredibly useful tool. It is even referred to as genetic scissors because CRISPR allows scientists to edit the genes (the DNA) of organisms, from the smallest cells to an entire mammal. Because of its revolutionary impact on life sciences, the discovery of CRISPR as a genome editor was awarded a Nobel Prize in 2020.
Just for a moment, let’s imagine the awe and excitement that scientists must have felt when they discovered the unusual, repetitive DNA sequences in prokaryotes that eventually led to CRISPR discovery, or when three different teams worldwide realized that this repetitive sequence functioned as an immune system. Think about the feelings of elation and accomplishment experienced when scientists discovered it could be used as an efficient genome editor.
If you were fortunate enough to be on a team that contributed to various CRISPR discoveries or are utilizing the system to create innovative uses or ground-breaking medical treatments, we congratulate you and thank you for your work. Let’s take a moment to look back and remember how phenomenal CRISPR truly is and review the astonishing research it is being used for today.
A Brief History of CRISPR
Just to set the stage, we will look back on some of the milestones that brought CRISPR technology to its current usefulness.
In 1987, an unusual, repetitive DNA sequence interspersed with spacer sequences was discovered in E. coli. Within a short amount of time, similar repeated sequence patterns were noticed in a range of other bacteria and archaea. In 2002, Jansen et. al referred to this as clustered regularly interspaced short palindromic repeats (CRISPR), and this mysterious family of sequences found only in prokaryotes gained a name that became used by the scientific community. During this time, an additional family of specific genes were found to be strictly associated with CRISPR (1) and were eventually given the name CRISPR-associated (Cas) genes. These Cas genes code for Cas proteins.
In 2005, three separate research groups published the discovery that CRISPR functioned as an immune system in prokaryotes (2, 3, 4). This theory was clearly proven in 2007 (5): Bacteria and archaea use Cas proteins to capture sequences of invading viral or plasmid genetic material. Then, they add pieces of the DNA or RNA to their own genome to use as memory to guide the Cas proteins in the future. When the same type of virus infects the organism again, the CRISPR sequence in the invaded organism is transcribed to generate CRISPR RNA (crRNA). The crRNA guides the Cas protein to cleave complementary DNA or RNA viral sequences, which prevents the organism from being infected by the same virus multiple times.
Only five years after the discovery of CRISPR as an immune system that cleaves viral genetic material, Emmanuelle Charpentier and Jennifer Doudna published evidence that the CRISPR system could cut targeted DNA (6). They successfully recreated the bacterial genetic scissors and simplified the molecular components. Then, they reprogrammed it to cut DNA at a predetermined site and the DNA was cleaved in the exact place that Charpentier and Doudna had decided upon. These phenomenal results showed the potential to use the precise system for RNA-programmable genome editing. Seven months later, the Broad team led by Feng Zhang also published evidence that CRISPR could be used as a genome editor (7). Both Virginijus Šikšnys and George Church (8) and their teams also developed these ideas independently and published results around the same time.
This discovery has had a revolutionary impact on life sciences. As with many other extraordinary breakthroughs, astounding progress has been made in the nine years since Charpentier and Doudna published their findings. During the following year, many other papers showed that the platform functioned in a variety of cells and organisms (9). In less than a decade, this incredible tool has proven useful in an astonishingly wide array of applications (for research and therapies) and is being used in human trials to cure disease.
In 2020, the Nobel Prize in Chemistry was awarded to Emmanuelle Charpentier and Jennifer Doudna for developing CRISPR-Cas9 as a method for genome editing, which is often referred to as “genetic scissors.” “These genetic scissors have taken the life sciences into a new epoch and, in many ways, are bringing the greatest benefit to humankind” (10).
Unfortunately, a long-running patent battle has been fought over CRISPR-Cas9. While Jennifer Doudna and Emmanuelle Charpentier first published evidence that the CRISPR system could be used as a gene editor seven months before the Broad team that was led by Feng Zhang did, Doudna and Charpentier’s team did not show in the initial paper that the system worked in eukaryotic cells. The Broad team received several CRISPR-related patents beginning in 2014. Doudna and Charpentier’s team (referred to as CVC) claimed this was patent interference and a long legal battle began. A PTAB trial delivered a mixed verdict in ruling that the eukaryotic CRISPR and other uses of the genome editor were separate inventions. Under this ruling, uses of eukaryotic CRISPR are patentable by Broad, while CVC can patent other uses. CVC appealed the decision to a federal court and was denied, then filed new claims with PTAB gaining an opportunity to provide more evidence. While not yet completed, the intensity of this battle highlights the importance of CRISPR-Cas9.
A Short Explanation of How CRISPR Works As A Genome Editor
Engineered CRISPR systems work with an RNA molecule called guide RNA (gRNA) that finds a specific DNA sequence, and a DNA cutting component. Currently, the most commonly used cutting protein is known as Cas9, but others can be used and some will target RNA instead of DNA.
When CRISPR-Cas9 is delivered to a cell, it finds and binds to a 20-DNA-letter long sequence that matches part of the guide RNA sequence. Then, it cuts the cell’s genome at an exact, desired location. Incredibly, this can be done with great accuracy in living organisms because the gRNA sequence must be specific to the target DNA compared to the rest of the genome. This process then allows genes to be disabled, removed, added, or modified using a repair template.
© Johan Jarnestad/The Royal Swedish Academy of Sciences”
Prior to the CRISPR system, gene modification was difficult, expensive, and time-consuming (requiring many months of work). Now, it can be done in a matter of weeks. Due to the fact that CRISPR is comparatively simple and inexpensive, adaptable, fast, and precise, it has rapidly become the most popular genome engineering approach. Additionally, customized Cas proteins have been created to produce specific results. CRISPR activation (CRISPRa) turns genes on, CRISPR interference (CRISPRi) turns genes off, and base editors only change one letter of DNA code to another.
However, it is not perfect. There is room for error, because gRNA targeting sequences may have additional sites throughout the genome that are a partial match. When this occurs, it can inadvertently edit genes other than the desired one (off-target edits) which could cause serious health problems. This is a hurdle that must still be overcome.
CRISPR Has Already Been Entangled in Ethical Concerns
Despite the enormous potential to improve life and bring better treatments, this spectacular discovery has not been without its controversies. Most notable was the editing of human genes by the biophysicist He Jainkui in 2018, in a pair of twin girls shortly after fertilization. The girls, and an additional gene-edited baby, were born alive and appear healthy, but using CRISPR to change their DNA (with the hope of making them resistant to HIV infection) went against all current ethical rules on human gene editing and caused a global outcry because ethical standards were ignored. The fact that these mutations can be inherited by any children the girls have, potentially changing the human germ line, is another major concern. Additionally, as noted above, CRISPR gene editing can cause off-target edits, and the technology is still too new to know what unintended effect such an edit will have on the children.
Disabling the girls’ CCR5 gene was likely done with good intentions. Regardless of the intent behind the experiment, it was tried far too soon to ensure safety and without following regulations or medical and research ethical standards. As a result, a Chinese Court handed down prison sentences and fines to He Jainkui and two of his collaborators. The People’s Court of Nanshan District of Shenzhen stated that “He and two colleagues had flouted regulations and research and medical ethics by altering genes in human embryos that were then implanted into two women” (11). Additionally, the health ministry banned the trio from working with human reproductive technology, the science ministry banned them from applying for research funding, and they were condemned by the scientific community.
Prior to this case, gene editing in both viable and non-viable human embryos was already a topic of debate. At least four other teams had published papers describing experiments using gene-editing techniques in human embryos (12, 13, 14, 15). However, many in the scientific community were afraid that it would lead to the exact situation created by He, and CRISPR-Cas9 gene-editing technology is not yet advanced enough to be used for reproductive purposes.
This case prompted the World Health Organization to create a committee of experts whose purpose is to develop a governance framework that establishes global standards and oversight of human genome editing. The committee’s work is still in progress, but has determined that “it would be irresponsible for anyone to proceed with clinical applications of human germline genome editing” and that “regulatory authorities in all countries should not allow any further work in this area until its implications have been properly considered.”
Current Applications That Are Benefiting Humanity
CRISPR is incredibly useful in many areas and the range of applications it is already used for is truly astounding. It has been widely used in basic research, from biochemistry to genetics, and the understanding of diseases. CRISPR tools can be used by scientists to understand how genes function and interact (16), or for large-scale genetic screens. “It is easy to implement due to the simplicity of gRNA design, programmable for different genomic loci, capable of detecting multiple genomic loci, and compatible with live cell imaging” (17).
CRISPR-Cas9 can also serve as a potent therapeutic tool. Since it can cleave the genomes of pathogenic bacteria, it can be used as an antimicrobial agent (18). Medical research into cancer, viral infections, and genetic diseases has benefitted from the application of CRISPR. Here are just a few examples of promising developments in this area: it has been successfully used in mice to correct the mutation responsible for Duchenne muscular dystrophy (DMD) (19, 20) and to target genetic liver disease (21). It has been used in vitro to target hepatitis B virus (22), HIV-1 provirus (23), and human papillomaviruses in cervical carcinoma cells (24).
CRISPR is also highly useful as a diagnostic tool. As mentioned in a previous article discussing OTS members’ efforts against COVID-19, the novel CRISPR-based nucleic acid detection technique called SHERLOCK was the very first FDA-authorized use of CRISPR technology to detect SARS-CoV-2. A genome-wide CRISPR screen identified several host factors that regulate SARS-CoV-2 entry into the cell (25) and another revealed multiple host factors that are critical for SARS-CoV-2 infection (26).
Interestingly, besides medical applications, CRISPR technology can be used to develop innovative crops, including those that can withstand drought, cold, salt, pathogens, herbicides, and pests (9, 16, 27). Plant researchers have also used CRISPR to enhance yield (27, 28) and the nutritional value of crops (27, 29). “Genome editing with CRISPR-Cas9 is amendable to edit any gene in any plant species. Because of its simplicity, efficiency, low cost, and the possibility to target multiple genes, it allows faster genetic modification than other techniques” (27). CRISPR is utilized in producing other food products as well. We can now easily genetically alter food-grade bacteria (30) and enhance the outcomes of beneficial organisms which include starter cultures and probiotics, while minimizing detrimental organisms such as pathogens and microorganisms that cause spoilage (31).
Potential Life-Changing Medical Applications
We are just beginning to see the true potential of CRISPR in ground-breaking medical treatments. A benefit in treating disease with gene editing is that you are correcting the molecular defect, providing a cure rather than addressing the symptoms. Additionally, the concern seen in He Jainkui’s experiment is not a worry here because, unlike when editing genes in a human embryo, editing genes in an adult or child does not change every cell in the body. Therefore, it does not allow the person to pass on any mutations to their future children.
CRISPR shows great promise as a treatment for cancers and genetic diseases. Here are just a few ways that Scientists and Physicians are trying to use CRISPR Cas9 to improve people’s health.
- CRISPR Therapeutics and Vertex Pharmaceuticals announced the successful treatment of one patient with transfusion-dependent beta-thalassemia (TDT) and one with sickle cell disease (SCD) using CRISPR/Cas9 gene therapy, CTX001 (32). In this therapy, a patient’s hematopoietic stem cells are edited to produce high levels of fetal hemoglobin in red blood cells. They are now in phase 1/2 trials.
- Editas Medicine and Allergan are in a phase 1/2 trial to test EDIT-101, to correct a mutation that causes Leber congenital amaurosis (LCA) type 10, a retinal degenerative disease. It is the first trial using a CRISPR agent inside the human body (33).
- Intellia Therapeutics treated their first patient in November 2020 with a therapy utilizing CRISPR-Cas9 that potentially halts and reverses transthyretin amyloidosis (ATTR) progression.
- A different clinical phase 1/2 trial is utilizing an infusion of hematopoietic stem cell transplantation (HSCT) using CRISPR/Cas9 edited red blood cells to treat Sickle Cell Disease.
- A study is determining the safety and feasibility of transplantation with CRISPR/Cas9 CCR5 gene-modified CD34+ hematopoietic stem/progenitor cells for patients that develop AIDS and hematological malignancies.
- A phase I study is evaluating treatment of CRISPR-Cas9 mediated PD-1 and TCR gene-knocked out chimeric antigen receptor (CAR) T cells in patients with mesothelin positive multiple solid tumors.
- CRISPR-Cas9 technology was used to design nanobody-based chimeric antigen receptor (CAR) T cells to treat solid tumors. This method “displayed potent cytotoxicity towards CD105+ tumor cell growth in vitro, and significant inhibition against solid tumors via specifically targeting human CD105 on tumor and tumor vascular endothelial cell of the microenvironment in the xenograft mice models” (34).
- Targeting herpes simplex virus (HSV-1) with CRISPR–Cas9 cured herpetic stromal keratitis, which causes blindness, in mice and inhibited viral replication in human-derived corneas without causing off-target effects (35).
- Caribou Biosciences, which was co-founded by Jennifer Doudna, has treatment for a form of non-Hodgkin lymphoma in Phase 1 clinical trial and is researching therapies for multiple myeloma, acute myeloid leukemia, and solid tumors.
Looking Toward Future Discoveries
The development of CRISPR into a gene-editing tool has revolutionized the way we approach many areas of research, innovation, and medical treatments. In the coming decades, it will be fascinating to watch as developments that we have not yet imagined are made possible through the use of CRISPR. It is likely that the discoveries we have already found using CRISPR will prove to be a small fraction of the total and CRISPR will long be a tool through which we gain the joy of discovery and provide untold benefits to humankind.